Research
In electrochemical devices such as lithium-ion secondary batteries, various phenomena occur during operation (electrochemical reactions), including charge transfer at the electrode/electrolyte interface, charge compensation and phase transition of electrode materials (active materials), and reaction distribution due to electron and ion transport resistance within the composite electrode. Since the properties of electrochemical devices are influenced by these phenomena, their understanding is essential for the development of high-performance electrochemical devices. In our laboratory, we are working on understanding the electronic structure of electrode materials in particular and designing new materials utilizing the knowledge obtained from the understanding by making full use of (operando or in situ) analysis under electrochemical reaction conditions using synchrotron radiation X-rays.
Please see below for specific research.
Elucidation of reaction mechanisms at the electrode/electrolyte interface in electrochemical devices
Since the electrode and electrolyte each have different internal potentials, potential compensation occurs at the electrode/electrolyte interface, resulting in an electronic structure different from that of the bulk. However, the electric double layer formed at the electrode/electrolyte interface is difficult to analyze because it is on the nm order. In convention, interface reaction analysis has been limited to methods such as DC polarization measurement and impedance measurement, which measure the relationship between reaction rate (current) and energy (potential) in electrode reactions, and combine this with post-measurement cell disassembly analysis to infer the reaction mechanism. Therefore, information on the interface structure during electrochemical reactions (non-equilibrium state) was critically lacking. Therefore, we have succeeded in observing the electronic and local structures of the electrode/electrolyte interface on the nanometer order by operating observation of the electrode/electrolyte interface under electrochemical reaction conditions using a model electrode that can define the interface and synchrotron radiation X-ray analysis. In lithium-ion batteries, we have elucidated the formation of a unique electronic structure at the electrode/electrolyte interface, which is the starting point of degradation, using operando total reflection X-ray fluorescence absorption spectroscopy and depth-resolved X-ray absorption spectroscopy (Figure 1, J. Phys. Chem. C 2014, 118, 9538. ). Based on this knowledge, we have also shown that the interface structure of electrodes can be stabilized by surface control (Electrochemistry 2014, 82, 891.). Further developing these methods, we have used newly developed operando soft X-ray absorption spectroscopy in magnesium secondary batteries to elucidate that the strong electrostatic interaction between anions and Mg2+ in the electrolyte makes Mg2+ coordinate to the anions, resulting in that Mg2+ decomposes on the anode surface to inhibit the reaction (Figure 2, ACS Appl. Mater. Interfaces 2020, 12, 25775.). Based on this knowledge, we have found that when the electrostatic interaction with Mg2+ is weakened by using huge anions, the Coulombic efficiency of precipitation and dissolution of magnesium metal is dramatically improved, and the high oxidation limiting potential makes it applicable to high-potential oxide cathodes (J. Phys. Chem. C , 2020, 124, 52, 28510 – 28519, Chem. Mater . 2021, 33, 1006.).
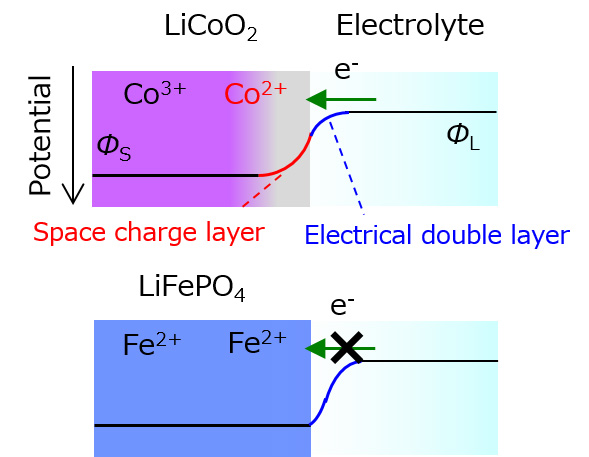
Figure 1 J. Phys. Chem. C 2014, 118, 9538.
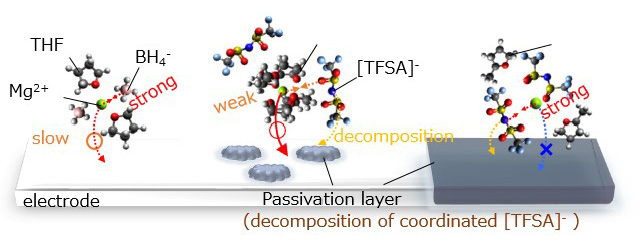
Figure 2 ACS Appl. Mater. Interfaces 2020, 12, 25775.
Development of high performance energy storage device electrodes based on elucidation and control of anion electronic structure
In the electrode reaction of energy storage devices, the capacity is determined by the amount of charge compensation of the electrode material due to the movement of carrier ions, so it is important to elucidate and control the electronic structure of electrode materials. Although studies on the elucidation and control of the electronic structure of electrode materials have been conducted vigorously, focusing on transition metal cations, the development of electrode materials through the control of transition metal cations has reached its limit. Therefore, we focused on charge compensation of anions instead of transition metal cations in electrode materials and established a guideline for material design by anion control by elucidating the mechanism using operando soft X-ray absorption spectroscopy. For lithium-rich oxide cathode materials in which charge compensation of oxide ions occurs during insertion and extraction of lithium ions, we found that the oxide ions are responsible for reversible charge compensation when either the ionic or covalent bonding between the transition metal cation and oxide is extremely strong, using operando soft X-ray absorption spectroscopy(Figure 1 Chem. Mater. 2020, 32, 1, 139.). Based on these findings, we developed a high-capacity electrode material by stabilizing charge compensation of oxide ions in lithium-rich oxide cathodes by controlling anions (Figure 2 ACS Appl. Energy Mater., 2020, 3, 5, 4162.).
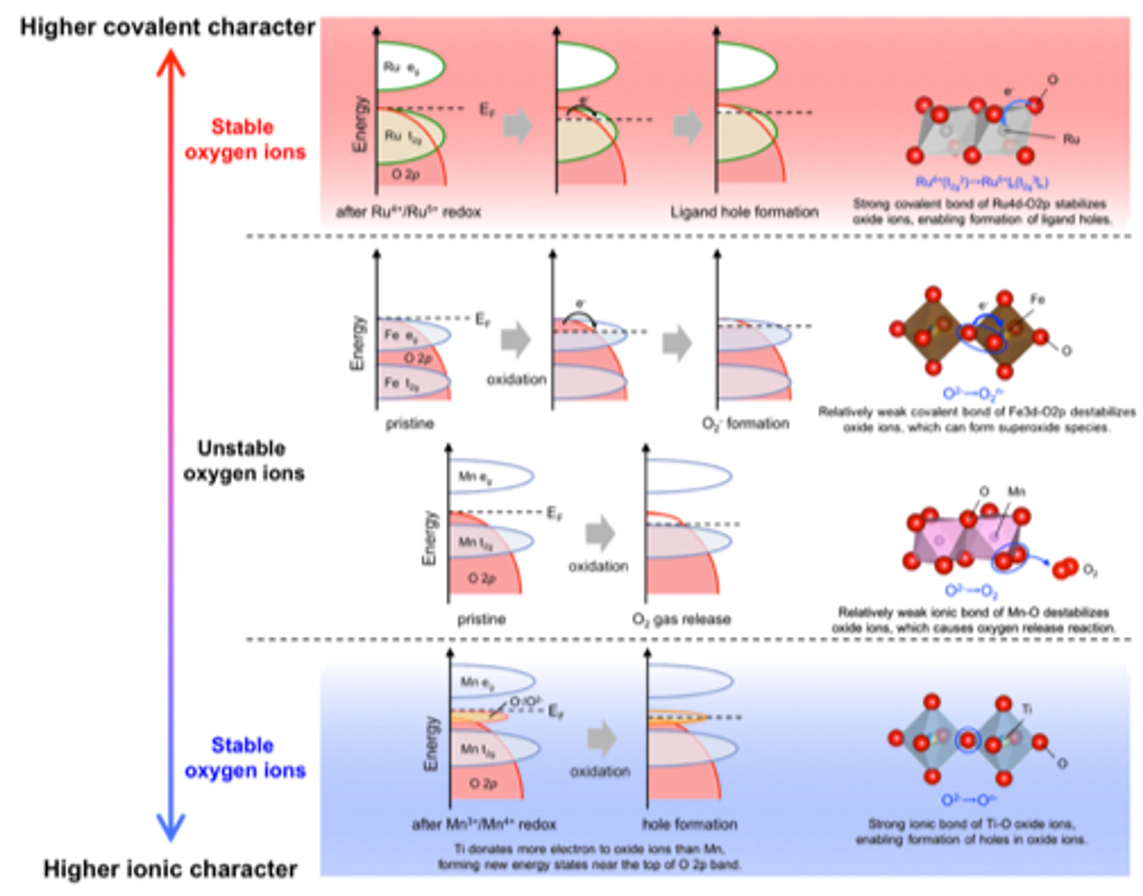
図1 Chem. Mater. 2020, 32, 1, 139.
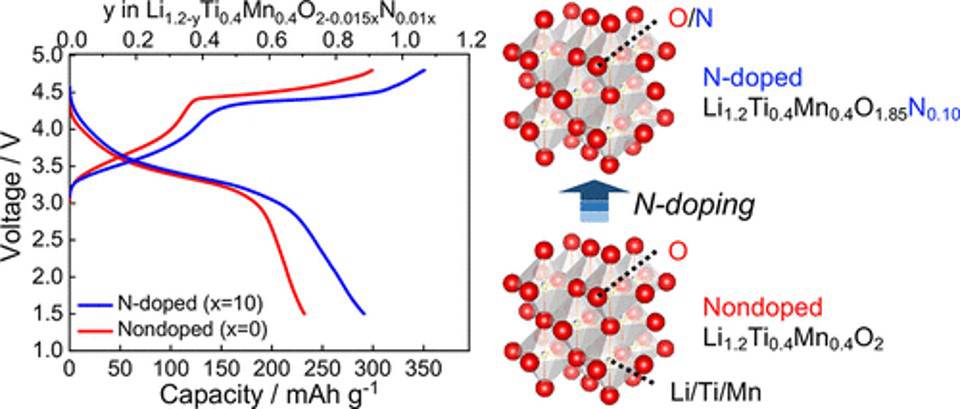
図2 ACS Appl. Energy Mater., 2020, 3, 5, 4162.
Material design of next generation battery with anion as carrier
We are developing materials for novel batteries that use anions as carriers, aiming to develop post-lithium-ion secondary batteries that surpass lithium-ion secondary batteries by using the knowledge gained through the elucidation of phenomena at the electrode/electrolyte interface and in electrode materials in lithium-ion secondary batteries. Fluoride ion rechargeable batteries, which use fluoride ions as carriers, are attracting attention as next-generation storage batteries because of their theoretically high energy density and high power density. Conventional research has focused on metal/metal fluorides such as Cu/CuF2 as cathode materials, but due to the rapid decrease in electronic conductivity and large volume change during fluorination and defluorination, there are issues of poor power density and cyclability. To solve this problem, we are researching cathode materials that utilize the intercalation reaction of fluoride ions. For example, we have found that replacing some of the oxide ions in Ruddlesden-Popper type oxides with fluoride ions, which have a perovskite analogous structure, suppresses phase transitions during charging and discharging, resulting in high power density.(Figure Chem. Mater. 2022, 34, 609.)。